Rapid, accurate characterisation of foodborne Salmonella isolates with nanopore sequencing
- Home
- Rapid, accurate characterisation of foodborne Salmonella isolates with nanopore sequencing
Foodborne illnesses are responsible for the loss of 33 million lives each year globally. Salmonella spp. bacteria are responsible for one of the four leading causes of diarrhoeal foodborne illness worldwide, and represent a major threat to public health1. Efficient and rapid surveillance of Salmonella spp. contamination within the supply chain, or during food production, is crucial to mitigate potential public health and economic burden.
Detection of Salmonella spp. has traditionally involved the time-consuming and labour-intensive process of serotyping to detect and distinguish among the 2,600-plus serotypes of Salmonella. To overcome these limitations, whole-genome sequencing (WGS) has been utilised more recently for tracking and surveillance of Salmonella. In addition to providing serotype data for isolates, WGS can characterise the antimicrobial resistance (AMR) genes within each isolate, information that traditional phenotype-based serotyping cannot provide.
The ability of nanopore sequencing to provide rapid, near-finished bacterial assemblies makes it an ideal tool for surveillance and AMR analysis of Salmonella isolates.
Rapid isolate sequencing
In a new study, Wu et al. from the Mars Global Food Safety Center in Beijing and the University of Georgia and Cornell University in the U.S. used nanopore sequencing for analysis of 69 Salmonella isolates, representing a mixture of common and rare Salmonella serotypes2.
DNA libraries were prepared using the Rapid Barcoding Kit and sequenced with the Oxford Nanopore GridION device. The authors commented that this multiplexed approach led to a 23% reduction in the cost of sequencing per isolate and allowed the team to analyse five samples at a time in just seven hours.
For serotype identification, raw nanopore sequencing data and assembled contigs were ported into the serotype prediction tool SeqSero2, and then only assembled contigs were analysed with SISTR, the Salmonella In Silico Typing Resource3,4. Wu et al. tested three depths of genome coverage — 15x, 30x, and 50x — and found that accuracy was nearly perfect with at least 30-fold coverage. ‘Accurate serotype prediction with [Oxford Nanopore] WGS data was achieved within about 5 h … at a minimum depth of Salmonella genome coverage at 30x’2.
AMR analysis
For antimicrobial resistance information, the team used assembled contigs produced from the serotype prediction workflow with two tools, RGI and Abricate5,6. They also tested precision and recall rates for various genome coverage depths. A single isolate representing the Typhimurium serotype was evaluated for AMR detection at 15x, 30x, 50x, 75x, and 100x genome coverage. With the tool Abricate , authors were able to perform a detailed characterisation of the AMR and virulence genes present and achieved accurate AMR prediction at a 30x depth of coverage.
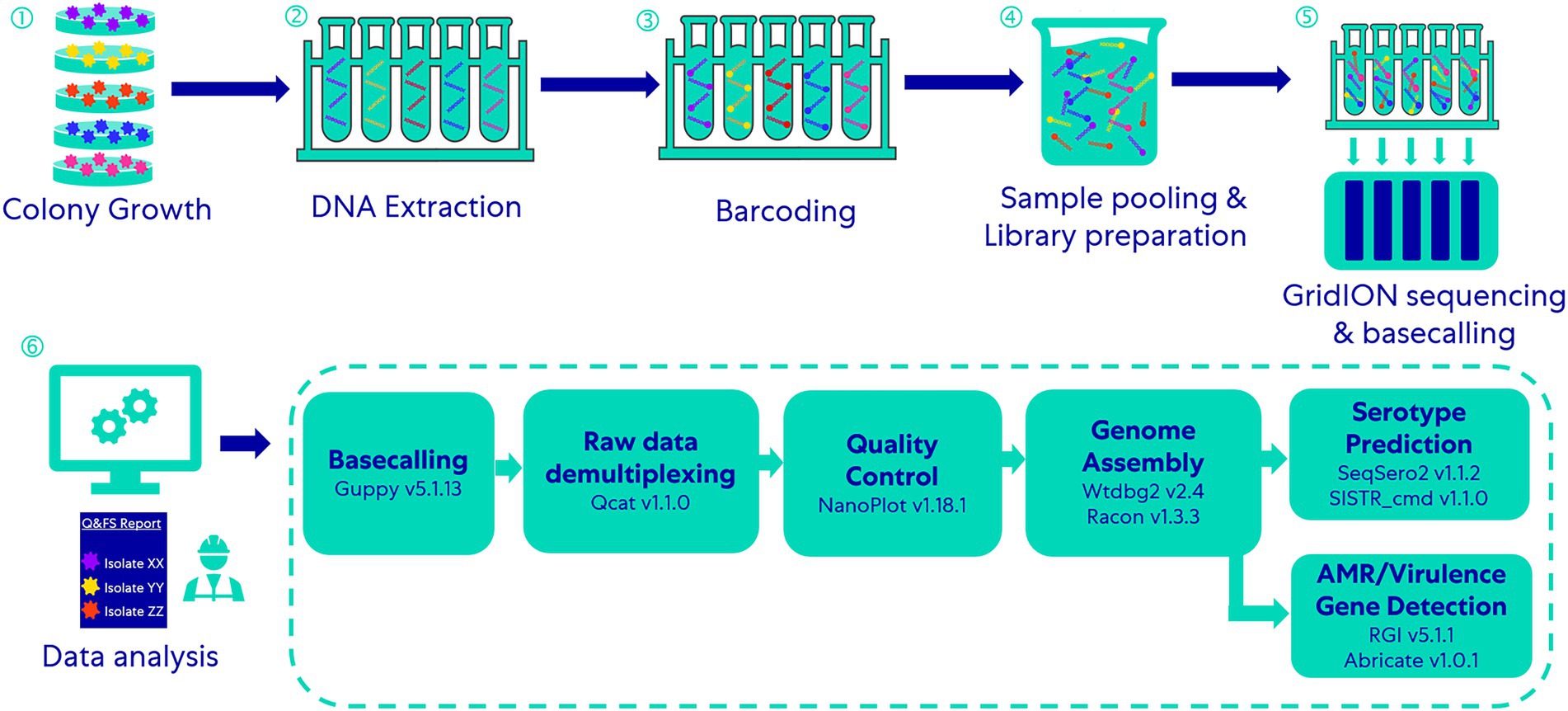
Importantly, all of these results could be generated rapidly from the sequence data. The authors commented that the nanopore approach ‘allows for Salmonella serotype prediction and AMR/virulence gene detection to be completed within one working day’ — a substantial improvement compared to conventional serotype testing, which can take as long as 12 days7.
This project provides a clear demonstration that multiplexed nanopore sequencing represents a reliable, accessible, and significantly faster alternative to conventional isolate testing in food safety laboratories — with the added benefit of simultaneously identifying serotype and characterising AMR genes in multiple Salmonella serovars with a single test. ‘This study paves the way for the application of nanopore sequencing in surveillance, tracking, and risk assessment of Salmonella across the food supply chain’, Wu et al. concluded2.
1. WHO (20 February 2018). Salmonella (non-typhoidal) Available at: https://www.who.int/news-room/fact-sheets/detail/salmonella-(non-typhoidal) [Accessed: 12 June 2023]
2. Wu, W. et al. Front. Microbiol. 13, 1073057 (2023). DOI: https://doi.org/10.3389/fmicb.2022.1073057 (2023)
3. GitHub. SeqSero2. Available at: https://github.com/denglab/SeqSero2 [Accessed: 12 June 2023]
4. GitHub. SISTR. Available at https://github.com/phac-nml/sistr_cmd [Accessed: 12 June 2023]
5. CARD. RGI. Available at https://card.mcmaster.ca/analyze/rgi [Accessed: 12 June 2023]
6. GitHub. Abricate. Available at https://github.com/tseemann/abricate [Accessed: 12 June 2023]
7. Xu, F. et al. Food Microbiol. 89, 103452 (2020). DOI: https://doi.org/10.1016/j.fm.2020.103452 (2020)